Reefkeeping: lighting research
Since I decided to start a saltwater tank a few days ago, I’ve been researching supplies and equipment constantly. There are so many options before even considering the vast species of corals, fish and other animals!
I found a solid introduction to reef tanks on animal-world.com, as well as a setup guide on Fishlore. Those helped me select substrate, opt for a protein skimmer and forgo a filter and understand the other equipment such as heaters and underwater fans called powerheads. Researching sumps and refugiums, which are secondary tanks, took a full day, as the decisions involved with plumbing the water between tanks are complicated. Lastly, I needed to research lighting.
There are many aquarium lighting products on the market, and many thorough guides are available online. Since I have a bit of an obsession with Arduino controlled LEDs, I figured I would create some custom lights for the tank. I wanted to be sure it would work, though, physically, so I needed to review some physics and biochemistry concepts in addition to reading about corals specifically.
How corals use light
Many corals and other invertebrates can derive energy from light indirectly via endosymbionts called zooxanthellae. These are single-celled dinoflagellates, a type of protozoan, that are able to live within the parent cell’s cytoplasm and range in color from a golden-yellow to brown. They are similar to mitochondria in animal cells and chloroplasts in plant cells, but those endosymbionts have shed many genes and are dependent on the host cell, whereas zooxanthellae exist both independently with two flagella and inside host cells after metamorphosis into a spherical form without flagella. Organisms can acquire zooxanthellae from sexual reproduction or from the environment by ingestion or other means. Organisms can expel zooxanthellae in a process known as bleaching and can subsequently be recolonized. Many different species of zooxanthellae can be present in a single host cell.
Zooxanthellae contain photosynthetic pigments:
- chlorophyll a: absorption maxima around 429 and 659 nm
- chlorophyll c: significant absorption in 447-452 nm region with maxima around 444-452, 577-585 and 626-629 nm depending on subtype
- peridinin: absorbs blue-green light in the 470-550 nm range, maximum at 483 nm
- diadinoxanthin: must research more, but may “give rise to xanthophylls” which may absorb blue and ultraviolet light
I haven’t found absorption spectra graphs for the pigments chlorophyll c, peridinin or diadinoxanthin, but the following graph is for chlorophyll a and chlorophyll b. Note zooxanthellae apparently do not contain chlorophyll b and thus the absorption of chlorophyll b should not be relevant to corals.
How corals react to light
Corals can react to stronger light by expelling some or all zooxanthellae cells or reducing the amount of chlorophyll within those cells since photosynthesis byproducts can reach levels toxic to the host cell. Similarly, the number of zooxanthellae cells and amount of chlorophyll they contain will increase under low light conditions. Since zooxanthellae are yellow or brown, their density affects the color of the coral such that corals become more brown with decreasing light intensity.
Corals may react to a change in wavelengths of light, but I did not find specifics other than “a typical lighting system for a reef aquarium consists of 50% white light with a high Kelvin rating and 50% blue, actinic light. ” I imagine there may be light absorption differences among zooxanthellae species and their populations or activity may be regulated on that basis.
In nature, ultraviolet light waves (UV-A and UV-B) penetrate the ocean’s surface but are filtered out as the light travels through the water. Both UV-A and UV-B light waves have been found to cause destruction of DNA and RNA within coral tissue. In response, many corals have made adaptations to reduce the effects of these harmful rays. These corals developed protective pigments that are often blue, purple, or pink in color. Most corals that contain these pigments come from shallow waters where the amount of UV-A and UV-B light is higher than in deeper areas of the reef. (http://www.liveaquaria.com/PIC/article.cfm?aid=114)
While it might seem increasing UV light would be desirable to trigger those protective pigments, I will take the experts’ advice to protect corals from UV. Note corals may lose colorful pigmentation after being moved to a new environment that has less UV radiation. It may be the corals had bright pigments as protection, and they are no longer needed.
It does seem “black light” is safe for corals, while further enhancing their fluorescence, based on the below information about black light wavelengths and that some reef lighting products contain UV LEDs.
Ultraviolet radiation
The UV wavelengths absorbed by protein and DNA are centered around 230 and 260 nm, respectively, with almost no absorption of wavelengths longer than 300 nm, which is still shorter than 400 nm, the shortest light visible by humans.
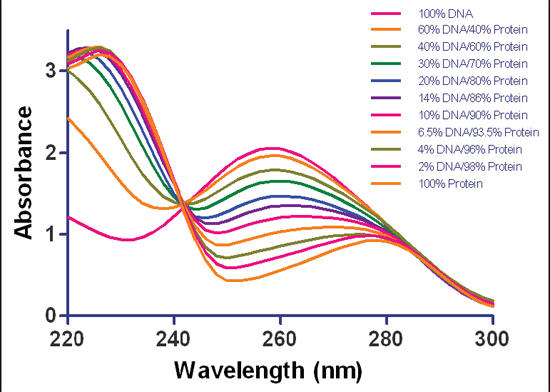
Absorbance spectral scans of purified dsDNA, protein or a mixture. Samples contained purified herring sperm dsDNA, BSA protein or a DNA/protein mixture at varying ratios (w/w). Measurements were taken at 1 nm intervals at wavelengths ranging from 220 to 300 nm. Data normalized to a 1 cm path length. (Brescia/BioTek Instruments)
“Black lights” emit mostly long-wave UVA radiation and, depending on the design, may emit little or no visible light. Ultraviolet LEDs are also available but are extremely inefficient below 365 nm and thus such UV LEDs on the market are generally around 395 nm.
Many substances, including within coral tissues, undergo fluorescence particularly strongly under UVA light, and that fluorescence may be in the visible range despite the light source itself being mostly invisible.
Since long wave ultraviolet, UVA, is 315-400 nm, it would seem UVA should not be destructive to DNA based on the above graph showing no absorption above 300 nm. That contradicts the above excerpt claiming UVA causes DNA and RNA destruction in coral tissue. In fact, UVA is now known to indirectly damage DNA by generating reactive chemical intermediates.
The DNA damage caused indirectly to skin by UVA consists mostly of single-strand breaks in DNA, while the damage caused by UVB includes direct formation of thymine dimers or other pyrimidine dimers, and double-strand DNA breakage. UVA is immunosuppressive for the entire body (accounting for a large part of the immunosuppressive effects of sunlight exposure), and is mutagenic for basal cell keratinocytes in skin. (Wikipedia)
Since “most sunburn happens due to UV at wavelengths near the boundary of the UVA and UVB bands”, I would bet a UV LED emitting light closer to 400 nm would not damage corals, but I didn’t determine what wavelengths of UVA were involved in the above described processes. Short wave ultraviolet, UVB and UVC, could clearly be destructive to cells, but such light would be produced only by specialty lamps and not consumer black lights (hopefully!).
Lighting goals
As could be surmised from the above information, there is a distinction in the aquarium community between total light and light relevant to corals. The following terms are used:
- Photosynthetic active radiation (PAR) is all light between 400 and 700 nm.
- Photosynthetic usable radiation (PUR) is the light that is actually used, which is basically a subset of PAR that emphasizes the blue and a bit of the red parts of the spectrum, corresponding to the wavelengths most readily absorbed by the zooxanthellae.
An extensive forum post describes the community sense that blue is most important and other wavelengths, especially when combined to form white light, are mostly for display and should be minimized, especially since red can apparently speed growth of nuisance algae. The author runs his blue lights 10 hours during which he runs his white lights 6 hours, though he mentions he could probably cut that down since he read “photosynthesis in corals only operates for 4-6 hours”. I haven’t confirmed that, but it seems to me 10 or 12 hours is a good starting point after which observing coral health should inform shortening or lengthening the light cycles.
The post further describes the need to change lighting slowly over the course of weeks, if lighting is to be changed, so the marine life can acclimate. This idea matches the above biological explanation of how the cells’ zooxanthellae might be expelled or change over time to match conditions.
White light
The Sun basically emits all wavelengths from 250 nm to beyond 2 μm according to Planck’s law. The same is true for candles and incandescent lamps. All of these can produce light that appears white or yellowish, depending on temperature.
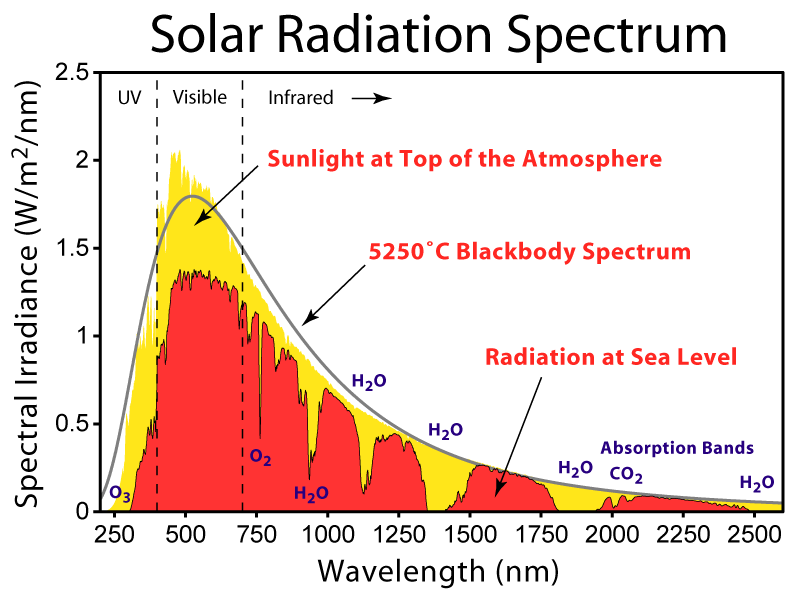
Solar irradiance spectrum above atmosphere and at surface (https://en.wikipedia.org/wiki/Solar_irradiance#/media/File:Solar_Spectrum.png)
There is no wavelength corresponding to white light, though, but rather it is a construction by human brains. Nevertheless, life on Earth evolved under this “broadband” white light, so it seems reasonable to try to replicate this type of light in products for plants and aquariums. Lamps producing apparently white light may not be the same full spectrum produced by incandescents or the Sun, though. For example, fluorescent bulbs employ a variety of designs that excite electrons to energies within consistent bands. Manufacturers aim to combine enough energies of resulting photons to emulate natural white light, but it can seem harsh, especially in cheaper bulbs.
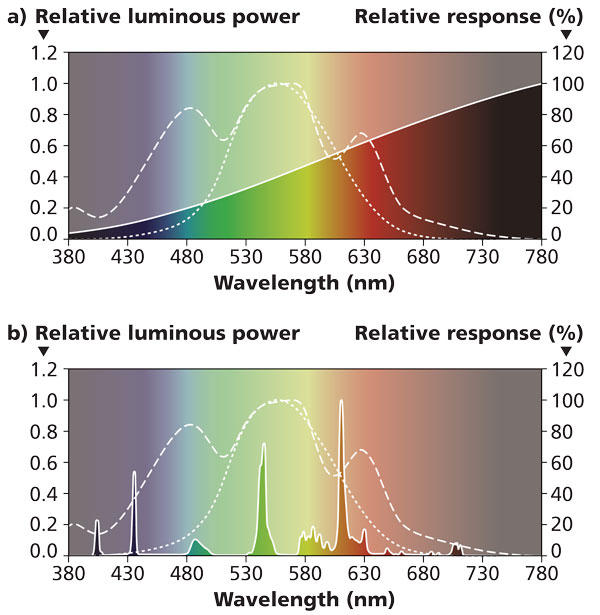
Looking at the color distribution of incandescent (top) and CFLs (bottom) in the charts above, we see that there are large sections of the full spectrum missing from each of these forms of white light. In incandescents, the lower wavelengths of blue, green all the way to yellow are found at a greatly diminished value. In CFLs, on the other hand, the apparent white light is something like a visual trick, composing narrow bands of violet, blue, green and orange color frequencies to trick our brains into thinking we’re seeing white light. (Source: LEDs Magazine) (flexfireleds.com)
Light emitting diodes are increasingly used as an energy efficient replacement for other lamps. LEDs have been made from dozens of inorganic semiconductor materials covering a range of wavelengths from ultraviolet to infrared. An individual LED generally produces close to a single wavelength of light determined by the band gap of the semiconductor material, but various modifications can be made to emit modulated (I think only longer) wavelengths. For example, “white” LEDs are commonly made using a blue or UV diode with a yellow or orange phosphor. This results in a spectrum covering a wider range than even white CFL bulbs, but still with a large “blue hump” that differs significantly from natural white light. Also, imperfections in the band gap width apparently cause the distribution of wavelengths centered around the target wavelength.
Newer LEDs employing “quantum dots”, known as QLEDs, may further address the narrow bands issue and produce more natural looking white light in the next few years.
Human vision
Humans see light via retinal rod and cone cells. Rods have an absorption maximum at 498 nm, in the blue region, but the cells aren’t involved with detecting color. Cones come in three varieties with absorption maxima at 437, 533 or 564 nm and detect blue, green or red light. Note the first and last of those maxima actually correspond to violet-indigo and green-yellow, but they absorb more blue or red light, respectively, than the green cones. The brain computes color from the data gathered by the three types of cones. Any given color humans perceive is thus not usually a direct measurement of that particular wavelength but rather a sort of interpolation based on the extent to which a light wave stimulates each of the three types of cones.
I do not know whether a single photon can stimulate multiple cones, but based on a cursory cone function review, I doubt it. Thus it seems a single photon of light could be perceived as any color, depending on the type of cone it stimulates, and useful color perception would probably depend on enough photons striking all three cone types such that a statistically valid determination could be made. Of course, a single photon would probably not be perceived at all, so perhaps this digression is inconsequential.
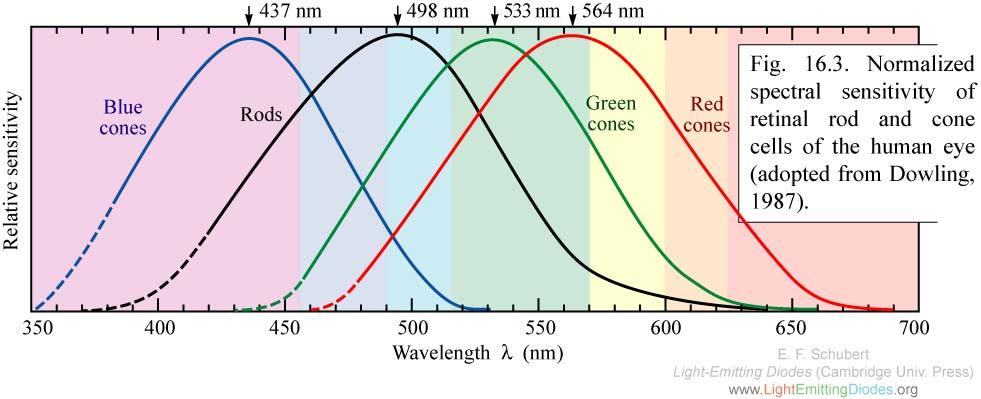
Human eye cone and rod sensitivity (https://iaincarstairs.wordpress.com/2011/07/26/the-willing-pupil)
Lighting products
Many commercial products exist based on LEDs, and they seem to be a combination of “white” LEDs and blue ones. Some products employ a variety of LED colors in an apparent attempt to fill in gaps in the spectrum, perhaps only for a pleasing appearance. A high end example is Ecotech Marine’s XR30w G4 PRO, which apparently uses eight types of LED. The spectrum graph seems pretty similar to a standard white LED but with a little more in the UV range.
Returning to the issue of actually lighting my tank, I need to decide whether the RGB LEDs I typically use in my projects will suffice for corals. The LEDs I use are Adafruit’s product, NeoPixels. I am a bit unsure of the exact underlying LED, though. Under “technical details” on the Adafruit NeoPixel Digital RGB LED Weatherproof Strip 60 LED -1m product page, the last three bullet points are:
- WS2812 Datasheet
- SK6812 Datasheet
- May ship with either WS2812B or SK6812-based LEDs. They are the same brightness, color and protocol
While it says they are the same color and brightness, that’s not true according to the datasheets, and especially if the LEDs can come with either WS2812 or WS2812B.
Chip | Wavelength (nm) | Luminosity (mcd) | |
---|---|---|---|
WS2812 specs | R | 620-630 | 550-700 |
G | 515-530 | 1100-1400 | |
B | 465-475 | 200-400 | |
WS2812B specs | R | 620-625 | 390-420 |
G | 522-525 | 660-720 | |
B | 465-467 | 180-200 | |
SK6812 specs | R | 620-625 | 700-1000 |
G | 522.5-525 | 1500-2200 | |
B | 467.5-470 | 700-1000 |
I’m not really sure what to make of that huge variation in luminosities or whether to believe “they are the same brightness”.
I also bought a strip of supposedly UV LEDs on Amazon (ACLOROL 5M 300 SMD5050 LED Strip UV Purple 395-405nm) and will experiment with using them with a tank. The product page does not list specific specs other than it is a 5050 chip (basically a physical size indication) and it is 395-405 nm, if that’s to be believed. Based on that and looking at the Wikipedia list of UV LED types, they are likely indium gallium nitride (InGaN) based, but it also would not surprise me if they are just blue LEDs with some filter attached. We will see. The product reviews are pretty bad, at any rate, with people claiming the strip got weak or broke within days or weeks.
So, I think I will go with my RGB LEDs to make some sort of light fixture and program controlled by an Arduino. I’ll mostly use blue with a bit of red, and possibly add in the UV LEDs, to try to match that higher end product’s spectrum. It may be worth incorporating some “white LED” product as well, but I’m aiming for simplicity (yeah, right).
References
- Zooxanthellae (Wikipedia)
- Factors that Influence Coral Coloration (LiveAquaria.com)
- Chlorophyll absorption and photosynthetic action spectra
- The Willing Pupil (ScienceAndReligion.com)
- Micro-Volume Purity Assessment of Nucleic Acids using A260/A280 Ratio and Spectral Scanning
- UV & Infrared Light (unitednuclear.com)
- Ultraviolet (Wikipedia)
- Discussion of light, lighting and how coral & zooxanthellae use light (reef2reef.com forum)
- LED Backlighting for LCDs Requires Unique Drivers (powerelectronics.com)
- Light Absorption for Photosynthesis (HyperPhysics, Georgia State University)
- Chlorophylls and Carotenoids: Measurement and Characterization by UV-VIS Spectroscopy
- Do’s & Don’ts with LED Aquarium Lights (LiveAquaria.com)
- LED lighting guide: saltwater (LiveAquaria.com)
- LED lighting guide: freshwater (LiveAquaria.com)
- Radion G4 (Ecotech Marine)
- Benefits Of The LED Color Spectrum (blog.flexfireleds.com)
- Solar irradiance (Wikipedia)
- Light-emitting diode: Colors and materials (Wikipedia)
- Quantum Dots Warm Up LED Lighting (photonics.com)